UMD-led MURI Findings Shed Light on the Coupling Between Electrical, Biochemical and Biomechanical Signals in Cells
Scientists have known for decades that electrical signals send messages to cells to tell our bodies what to do. New research published by teams in a Multidisciplinary University Research Initiative (MURI) led by the University of Maryland sheds light on additional biomechanical signals that might be used to tell cells what to do, such as help a wound heal faster.
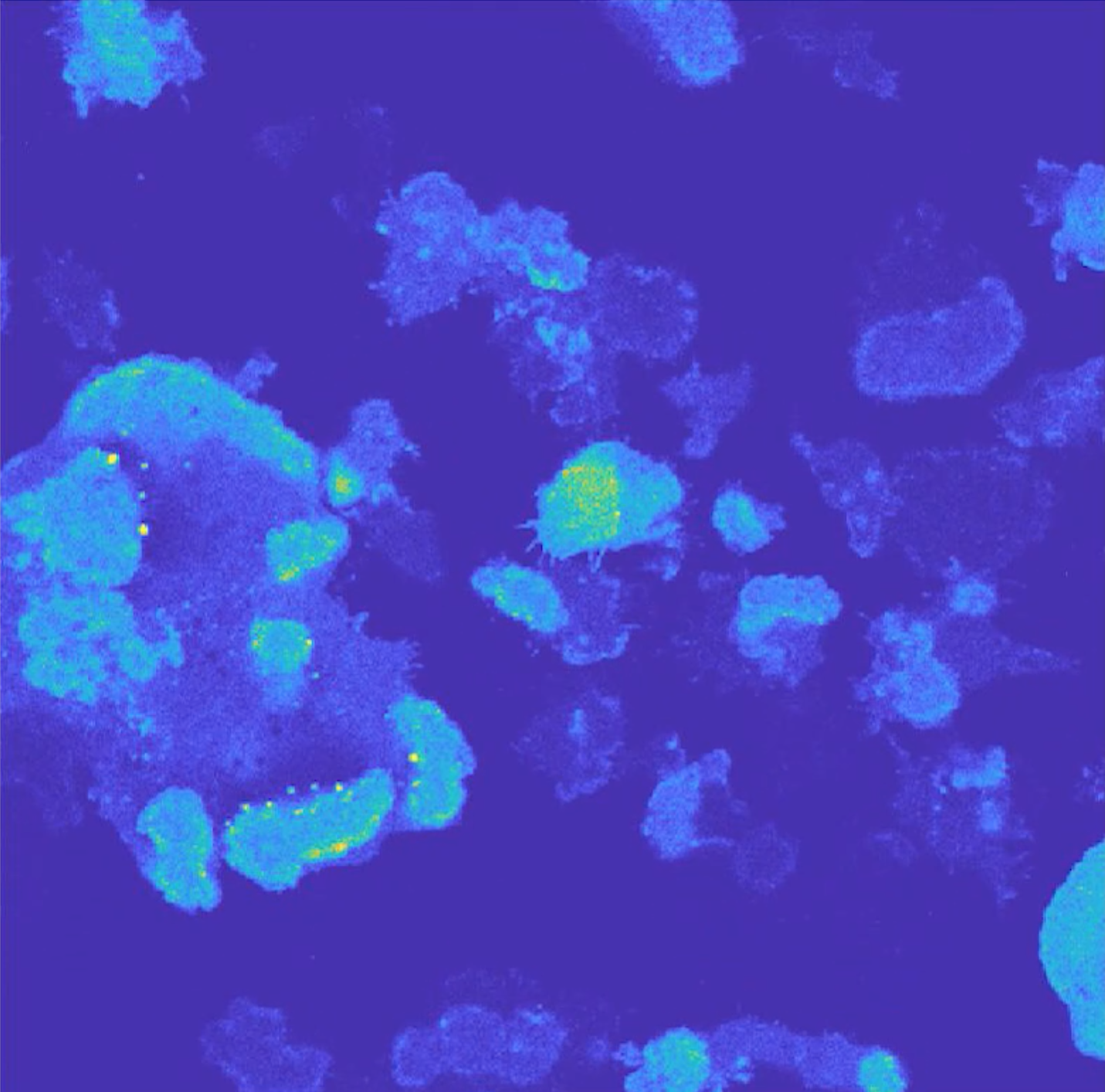
“Our research shows for the first time that biomechanical waves are always occurring inside cells and that they can act as sensors of the physical environment,” said Wolfgang Losert, a professor of physics at UMD and principal investigator of the MURI.
Two papers published in the journals iScience and eLife report recent findings of the MURI. Made up of physics, chemistry, biology, bioengineering and dermatology researchers from UMD and several other universities, the MURI team is trying to understand the coupling between electrical signals and biochemical and biomechanical signals.
“These two papers really highlight how they're connected,” said Losert, who also has a joint appointment in UMD's Institute for Physical Science and Technology. “This work opens up new avenues for steering cells and tissues and modulating cell fate with physical control inputs.”
The study reported in iScience shows that electric current sends out signals, like a Morse code, that a cell reads and reacts to.
“Our iScience study shows there is information encoded in the time sequence of signals and that the cells can read out the time sequence of the AC electric field. That impacts the fate of the cell,” Losert said. “The eLife paper shows that the cell’s sensor of the electric field is not a chemical concentration directly, but a dynamical system of internal waves and oscillations.”
Always on the Move
“Cells are constantly moving in your body, and that's really important for all kinds of processes. Like when you cut yourself, the wound closes,” explained Peter Devreotes, a member of the MURI team and a professor in the Department of Cell Biology at Johns Hopkins University. “One of the most important things for medicine is in cancer metastasis. When you have a tumor, the cells migrate and move out of the tumor to make a secondary tumor. So, cell movements are very important, and they can be guided. They can be guided by chemicals. And the other thing that's really surprising, that my entire career has been based on showing, is that they can also be guided by electric fields.”
In the iScience paper, the researchers focused on telling cells to become a different type of stem cell, which might be important in the body for medical treatment.
“People use cocktails of chemicals to tell the cells to become certain type of cell. And more recently, they use optogenetics to shine a light and induce the cells to become a certain type of cell,” said the iScience paper’s lead author Min Zhao, a professor of dermatology at the UC Davis School of Medicine. “Our MURI group has found that electrically we can regulate the cells to become certain type of cells and that electrically we can stimulate the cells to activate intracellular signaling.”
“We’ve understood that cells can be directed by chemical or light signals,” Devreotes added. “That’s a hard thing to apply in medicine, especially deep inside a tissue. Whereas an electric field has the potential of moving cells deep inside the body.”
Clever Approach
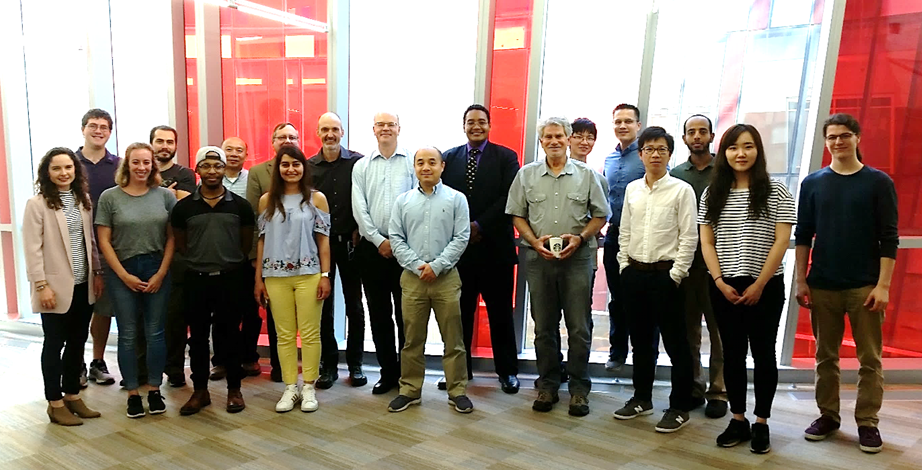
The MURI team’s study published in eLife brought new insights into how those electrical signals get to the cells, how long it takes and how cells react when they read the signals, a process known as excitability.
“In the electrical activity of the brain, it's well established that waves and oscillations are the thing we should look at when we try to understand how it processes information,” Losert said. “Now we're shifting the lens and saying when we're providing an input, we should also look at the time dependence of the input as potentially carrying information.”
A series of clever biological approaches led to the findings about how electric signals are sensed by cells. Those techniques were devised by MURI team member Qixin Yang (Ph.D. '22, physics).
“If you put cells in electric fields, they can move in accordance to the electric field. That's been known for a long time, but it's still kind of a mystery of how that happens,” Devreotes said. “What Qixin did was get closer to answering that by visualizing some of the molecules in the cells that we know make the cells move and seeing that those molecules were directly influenced by the electric field.”
“The cells are very small, such that you cannot distinguish the overall cell motion versus the subcellular dynamics,” Yang said. “You just don't have that resolution to study the subcellular dynamics independent of the cell motion.”
Building on an idea from Devreotes’ lab, Yang created a large canvas to get what she calls a giant cell.
“I fused tens of cells together using electric shock, such that you will have very large giant cells and a finer resolution to look at the subcellular dynamics,” Yang said. “With normal cells, what you see is like a fraction or fragment of this large canvas. But with giant cells, you will have that complete field of view.”
With this large canvas, the team set its sights on biomechanical waves as a potential novel sensor of electric fields. Losert, in collaboration with MURI team member and UMD Chemistry and Biochemistry Professor John Fourkas, identified these waves as likely sensors of surface topography, another physical input to the cells.
“Qixin showed that the biomechanical waves are also directly steered by electric fields,” Losert said. “She developed ways to quantify the duration of the waves in directions and discover that the electric field brings these waves closer to the threshold of excitability.”
The MURI team will continue to work to better understand the coupling between electrical signals and biochemical and biomechanical signals.
“These two papers really highlight how these signals are connected,” Losert said. “I think these two observations of information in the Morse code and sensing of electric fields by waves are novel and provide a framework for understanding which kind of device may work for directing cell movement.”
Losert credits strong collaborations between researchers at the different institutions with the MURI’s success so far.
“Five teams with different expertise came together in the MURI team to allow us to make this discovery,” Losert said. “It’s a new perspective on where information resides, what we should sense about living systems and how you might be able to actuate living systems.”
Written by Ellen Ternes