Mapping Molecules in the Brain
Decoding Disease, Targeting Threats
Though modern science has eradicated countless threats to human health, many other diseases and degenerative conditions continue to take a devastating toll. How can we eliminate these threats to health and well-being?
Researchers in the University of Maryland’s College of Computer, Mathematical, and Natural Sciences (CMNS) fight relentlessly against diseases and conditions that claim countless lives and undermine ability, function and perception. These scientists are committed to finding answers by mapping complex neurological circuitry to better understand how the brain works and what happens when something goes wrong, tapping into the power of disease-fighting T cells to stop cancer in its tracks, and unraveling complex mechanisms to better understand and treat neurological impairments caused by aging and disease.
Grand challenges demand bold ideas and real-world solutions. In CMNS, we lead Fearlessly Forward.
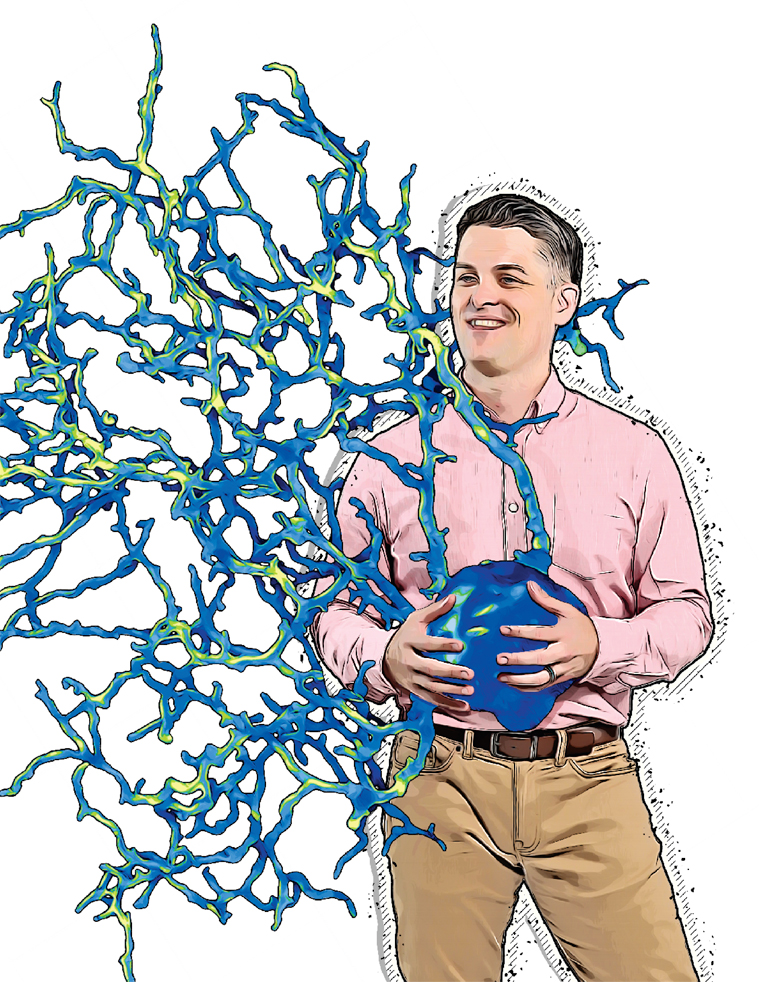
It’s hard to fix a machine you don’t understand. That means medical practitioners fighting brain disease and injury are at a disadvantage, because no one really knows how a human brain works. They don’t know how many cells it contains or how each cell connects with hundreds or thousands of others to form thoughts and control actions.
Biology Assistant Professor Colenso Speer has a plan to answer those questions. He creates innovative ways to map the physical layout of brain cells, known as neurons. And he uses sophisticated new techniques to identify the complex chemical signals between neurons that form the intricate circuitry in our brains.
“This research could help identify where and how things go wrong in the brain,” Speer said. “It could possibly lead us to genetic candidates for treating neuropsychiatric disorders, neurodevelopmental disorders, aging and memory disorders, and many other cognitive functions that are directly impacted by changes in the connections between neurons.”
Those connection points are called synapses, and they are extremely complex environments where thousands of different proteins create signals that flow from one neuron to another. These signals enable the brain to think and build memories.
“But proteins degrade very quickly, within hours to days,” Speer said. “So, how do our memories last for years? How do the neurons constantly produce new proteins to rebuild and maintain synaptic connections and keep our memories and capacity for learning alive?”
To find out, Speer and a team of UMD researchers are investigating neurons in the eyes to understand how they wire up to the brain during development and how synapses in visual circuits change in response to different visual stimuli. The team includes Cell Biology and Molecular Genetics Professor Najib El-Sayed and Chemistry and Biochemistry Professor Peter Nemes.
Together, they’re using transcriptomics—the study of RNA—to read the messages that neurons transport to their synapses in order to produce new proteins at the right time and place. Then, they apply advanced proteomics—the study of protein identities and abundances—to identify the exact proteins the synapses produce. They also use super-resolution microscopic techniques to image the physical arrangement of the molecules in synapses and map out how they organize as synapses develop.
Attacking the synapses from these three approaches will allow Speer and his colleagues to understand exactly how tiny molecules in the brain build and maintain its complex circuitry. The new tools they’re developing for this work will also help clinicians identify when this process goes wrong in a diseased brain.
The technical challenges of this work and its potential to transform scientific understanding of how neurons are wired earned Speer a $1.5 million National Institutes of Health Director’s New Innovator Award in 2020.
The risk is high because success is not guaranteed, but the reward would be an unprecedented list of parts and their interrelationships—at the level of RNA and proteins—in a developing synapse. And that would be a major step in understanding one of the most complex machines we know.
Written by Kimberly Cutlip
This article was published in the Fall 2023 issue of Odyssey magazine. To read other stories from that issue, please visit go.umd.edu/odyssey.
Also from this issue: